Anyone who has ever seen a floating leaf slide down a meandering river has noticed that when the water turns around a sharp corner, it creates small eddies, creating turbulence in contrast to the calm center of the flow. It may happen. Look a little closer and you’ll see how complex the turbulent flow is, stressing and abrasive the rocks and twigs it passes.
If you could peer into the bloodstream, you’d see the same thing at work, says Ian Tamargo, a medical student at Emory who recently earned his doctorate in molecular pharmacology.
“(Blood) flows forward, but when it hits a curved surface, it bounces back and rotates in a circle,” he says, explaining how the flow is intricately blocked when the blood hits a wall that blocks it from flowing. did. Straight line. “In the bifurcation region, it hits the bifurcation point directly and returns, creating an irregular flow pattern.”
You might imagine it when you think of a rafter trying to cross a rapid where the water flow becomes more dangerous. Like the flow of a fast-flowing river, the way our blood passes through critical points in our vascular system has its own potential for danger. Learning more about these stresses and their functions could help save lives through a better approach to heart disease, which the Centers for Disease Control and Prevention lists as the leading cause of death in adults.
This idea is the driving force behind the research of Tamargo’s leader, Dr. Hanjun Jo. He is a professor of medicine and biomedical engineering at Emory University and has spent more than 20 years developing new approaches to treating heart disease by understanding the complex dynamics of blood flow within this microscopic tissue. I’ve been searching. world.
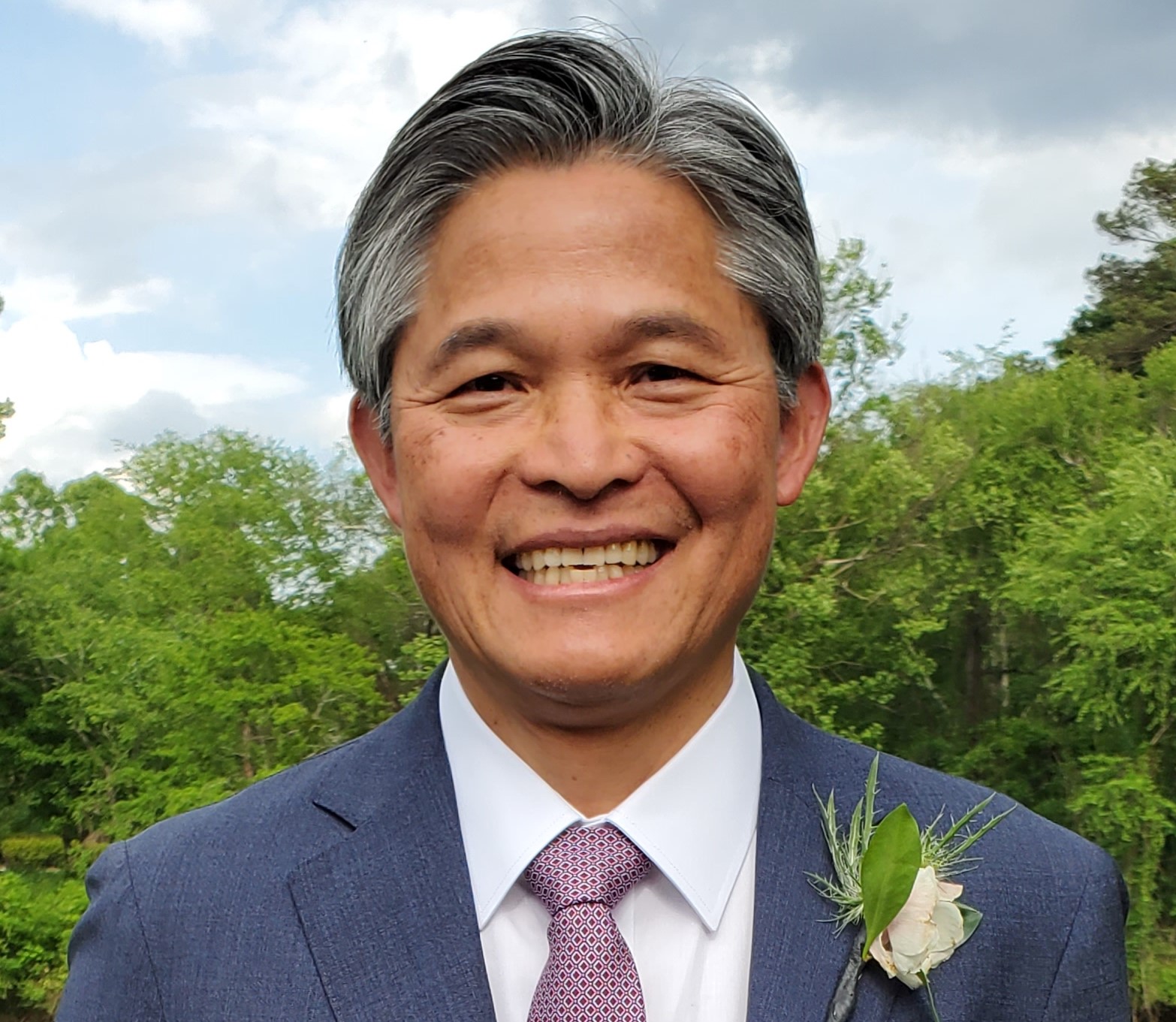
In the early 1990s, researchers in Joe’s lab discovered that when blood flows around curves, such as where one blood vessel splits into two, the tiny eddies and vortices are caused by specialized cells lining blood vessels. We discovered that it puts stress on the endothelial layer, which is a single layer. Regulates exchange between blood flow and other tissues of the body.
This disruption of blood flow changes the shape of endothelial cells, which are normally arranged in uniform strips like a “mown cornfield,” until they look like the disorganized cobblestones of an old street, says Tamargo. he says.
Increased stress not only changes the shape of endothelial cells, but also signals that something is terribly wrong and sends everything into red alert mode. This causes thickening of the blood vessel walls and atherosclerosis, the buildup of fat, cholesterol, and other substances (often called “plaque”) in and on the arterial walls. None of this is good news for people who have plaque buildup.
In contrast, in straight regions of blood vessels, blood typically flows in a gentle pattern, more similar to the center of a river than the edges. When that occurs, endothelial cell responses are likely to protect against atherosclerosis.
Atherosclerosis can be caused by many factors, including high cholesterol, diabetes, high blood pressure, a sedentary lifestyle, and aging. However, the blood flow stress that promotes disease is different in that it is not caused by external factors. Therefore, lifestyle changes do not affect the patient’s health.
Joe’s research aims to figure out how best to help patients experiencing heart blockages caused by this type of blood flow by harnessing the body’s natural systems to redirect and correct course. The purpose is
“There are many cases where exercise is not a practical solution,” he says. “By targeting flow-sensitive genes, we believe we can find new treatments for atherosclerosis.”
Over the past 15 years, Joe and colleagues have pioneered new technology that allows them to meticulously track hundreds of different stress pathways that increase the risk of plaque buildup in arteries.
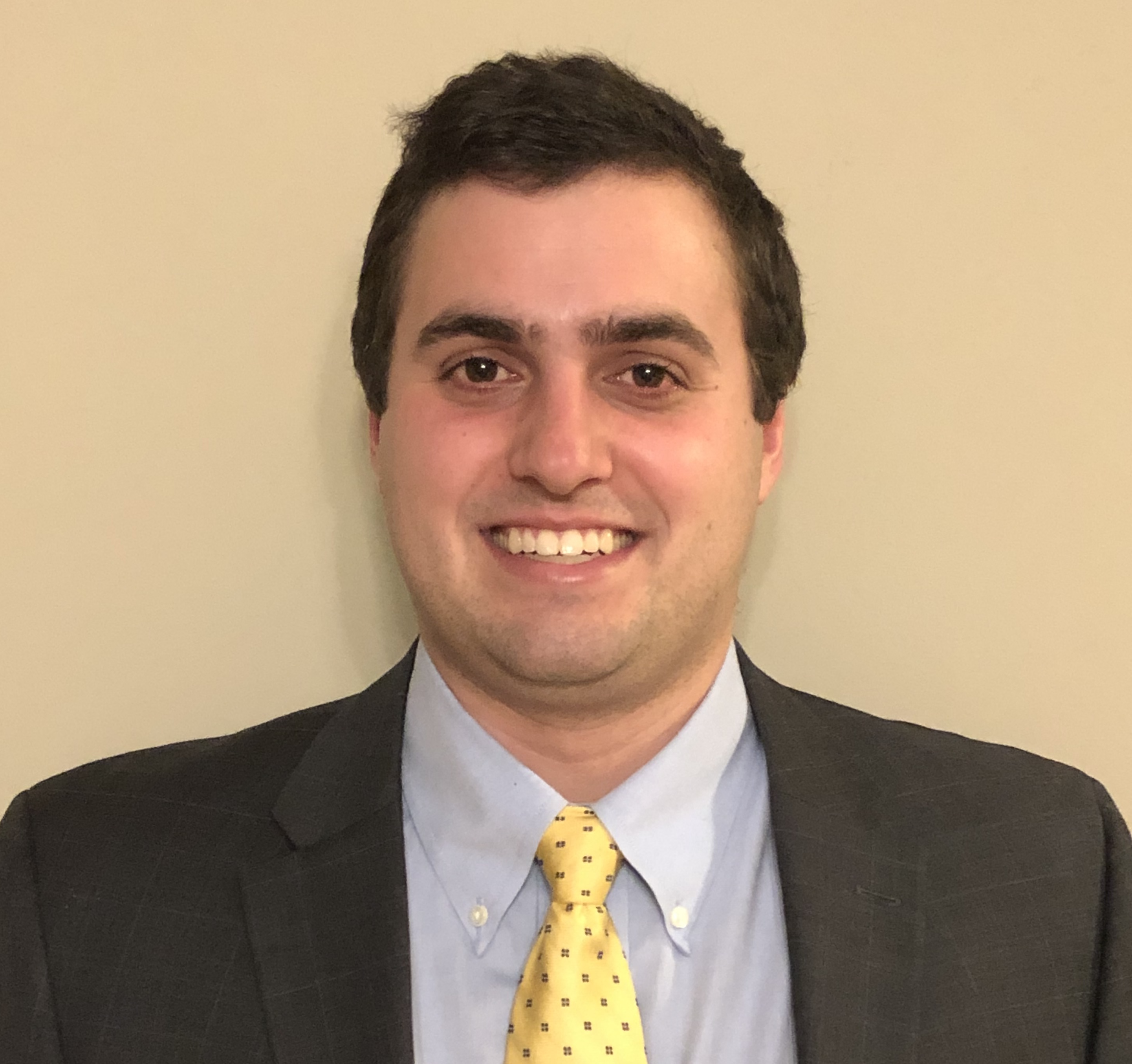
Researchers peered into the Petri dish and learned how to accurately measure the effects of different types of stress on endothelial cells under laboratory conditions. Using mice, we changed the state of blood flow in the left carotid artery from normal to turbulent, and the turbulent flow in the mice activated genes, causing endothelial inflammation in just one week, and atherosclerosis after one week. I was able to observe how it was caused. . Blood flow through the right carotid artery was left unimpeded and continued normally.
“By performing this simple surgery, we were able to demonstrate that impaired blood flow can cause atherosclerosis in animals with high cholesterol,” says Joe.
Once researchers observed how, they set out to figure out why. They found small fragments of proteins on endothelial cells that are sensitive to stress induced by turbulent flow. These proteins respond by initiating cell signaling pathways that activate genes.
“At the microscopic level, they almost act like windmills,” Tamargo says. “They accept mechanical forces called shear stress and transfer that energy into chemical activation.”
Over time, Joe and his collaborators created a catalog of thousands of genes sensitive to mechanical stress. “Different types of mechanical stress have different effects on gene expression,” Tamargo says. “And we can increase or decrease the production of proteins that specifically respond to those stress patterns.”
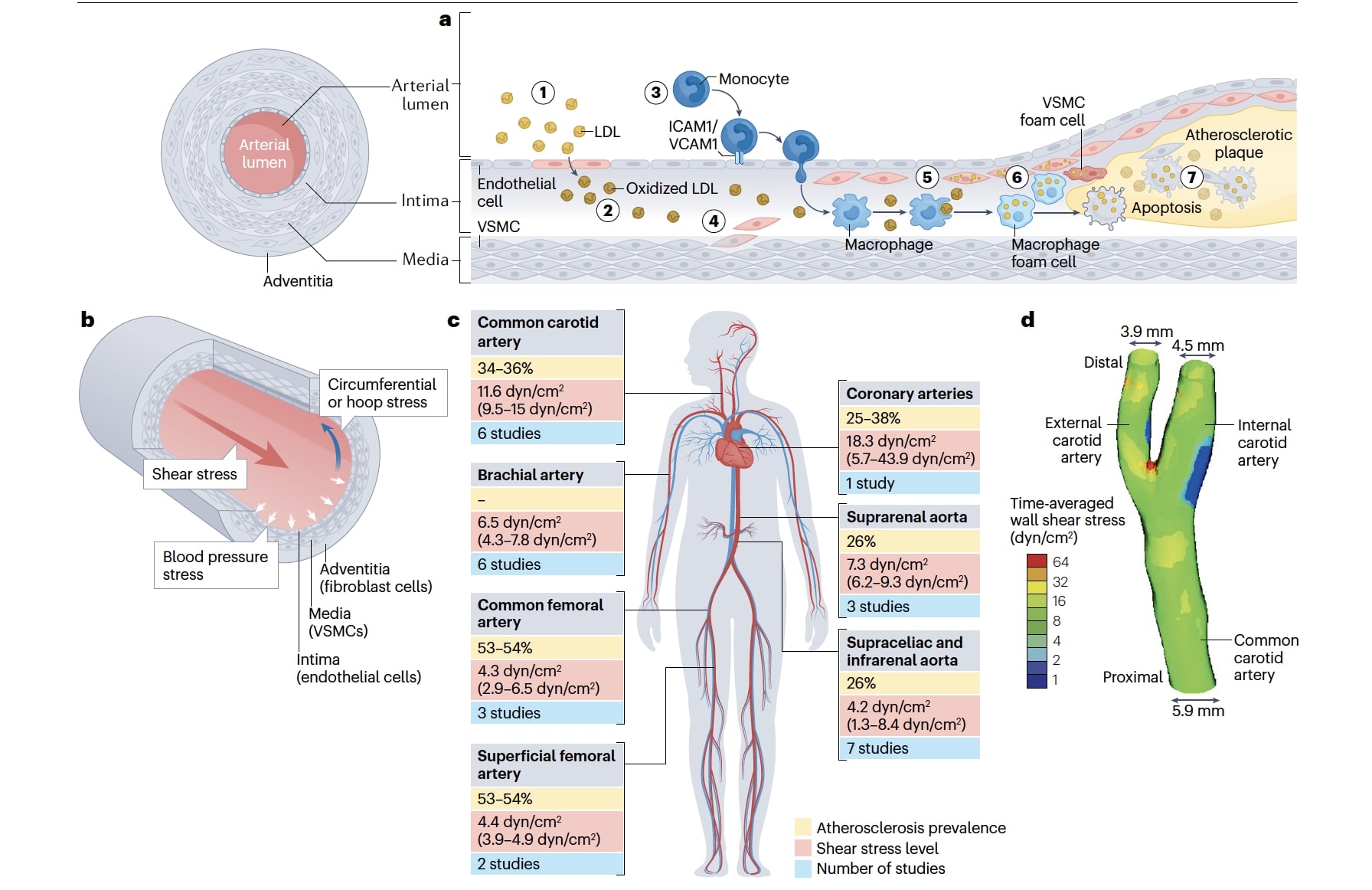
What will Joe, Tamargo, and their colleagues do next? We are developing new drugs and gene therapies to manipulate phlokine (also known as flokine). “It happens all the time,” Joe points out.
The hope is that they may ultimately lead to promising new treatments, such as alternatives to lipid-lowering drugs that reduce the risk of heart attacks and strokes. Joe encouraged me to 2017 clinical trial of anti-inflammatory drug canakinumabThis indicated that reducing vascular inflammation may play an independent role in reducing coronary events.
As someone who sits at the intersection of bioengineering and medicine in such a unique way, Joe enjoys working collaboratively across disciplines and using “engineering approaches to solving clinical problems.” He says he values his ability to conduct research.
“I wake up every day thinking that[this research]could do something very important for patients,” he says. “These diseases kill millions of people, but patients don’t wait. That’s why we’re excited about developing new treatments.”